Zooplankton swim, jump, and feed by beating cilia, flagella or appendages and strike frequencies are often so high, mainly for the smaller forms, that they cannot be resolved by the naked eye nor by ordinary video. Mechanisms of feeding and moving can often only be revealed by the use of high speed video.
Lucky Luke of the ocean: Ambush attacks in copepods
Copepods live in a viscous world. Imagine that you cannot get your drink out of the glass because the fluid is as thick as syrup, or that you reach out to get your Danish pastry just to realise that you push it away as you try to grab it. Filtering water for microscopic food particles or grabbing prey items become non-trivial tasks in a viscous environment. Many copepods are ambush feeders that in rapid attack jumps capture prey that swim into the dining sphere of the copepod. Below we see that this is possible despite the sticky nature of water at this scale (see Kiørboe et al. 2009).
In the mm-sized Acatia tonsa, the attack jump is accomplished by the 4 pairs of swimming legs that sequentially strike backwards and accelerates the copepod forward. The copepod then opens the feeding basket, made up of the feeding appendages, and suck in the prey. The entire process may be accomplished in less than 10 ms (=1/100 s).
Ambush attacks in the tiny copepod Oithona davisae are very similar to that observed in Acartia. The prey is captured by the 2nd maxillae and the maxillipeds that stretch out immediately after the forward lunge and the entire attack my be completed in less than 3 ms. The maximum speed during the attack is more than 100 mm/s, corresponding to > 300 body lengths per second, and the acceleration may exceed 100 m s^-2.
A striking feature of the attacks is that the prey is NOT pushed away as the copepod shoots towards it, as one would imagine in the viscous world of the copepod. This can be seen in the sequence of frozen video images in the upper row (prey is indicated by arrow, 0.5 ms between images), or in the lower row, where position of copepod and prey immediately before (in white) and after (in black) the attack has been indicated (from Kiørboe et al. 2009).
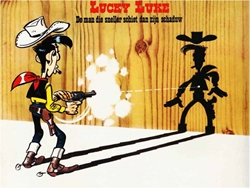
We have named the mechanism that allows the copepod to attack its prey without pushing the prey away the 'Lucky Luke Effect'. Lucky Luke is faster than his own shaddow. Similarly, the duration of the attack is shorter than the time necessary for the sticky boundary layer to develop around the copepod. The timescale of this process, the 'viscous time scale', is the distance (to the prey) squared divided by the viscosity of the waterIt is on the order of 10-100 ms, smallest for the smaller copepods. Thus, rapidity is the key to the success of this feeding strategy
The strongest animal in the world: copepod escape jumps
Pelagic copepods are believed to be the most common metazoans in the ocean and, maybe, on this planet. Their evolutionary success is due, among other things, to their capability of detecting approaching predators as well as their unique escape capability: on perceiving a predator, a copepod may accelerate to a velocity of near 1000 body lengths per seconds within a few ms and this way bring itself at safe distance from a predator. These velocites are 10 times higher than reported startle velocities for other similarly sized aquatic animals.
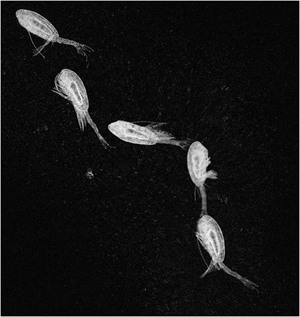
Escaping copepod (frozen high speed video images)
The mm-sized copepod Acartia tonsa performs an escape jump, shown in slow motion (1 s becomes ca. 5 min). The 4 pairs of swimming legs strike backwards sequentially, accelerating the copepod to a peak velocity of 500 mm/s; during the recovery stroke the copepod coasts while decelerating, and a new power stroke is initiated before the copepod stops. Many such consecutive stroke cycles may bring the copepod fast and far away from a predator.
Escape jumps of a small (0.3 mm) and a large (3 mm) copepod (Oithona davisae and Calanus finmarchicus). Note how the bigger copepod coasts between power strokes: the jump brings the copepod into a high Reynolds number regime, where inertia becomes important.
The force production required to achive these high acellerations and velocities varies between 500-1500 Newton per kg muscle mass. This is 10 times more than reported for any other animal motor. This is surprising, also because the peak performance of all other animal motors varies rather little around a value of 57 N/kg. Read more about this unique capability in Kiørboe et al. 2010.
Quiet swimming
Zooplankters move to find food and mates, but moving also implies an elevated risk of encountering a predator. A moving zooplankter generates hydrodynamic noise in the water, and such noise may be perceived by rheotactic predators. Like a submarine that aims at moving quietly, so should a zooplankter move without making too much noise. Zooplankters can either move by vibrating cilia, flagellae or appendages continuously, which results in a smooth translation; or they can move erratically by intermittently striking cilia or appendages.
SloMo movies of swimming copepods. Left: Metridia longicornis (2.5 mm) moves smoothly by continuously vibrating the feeding appendages. Right: Oithona davisae (0.3 mm) swims by intermittently kicking the swimming legs (swimming by jumping), resulting in a highly variable propagation velocity. Intuitively, one would expect erratic swimming to be both more expensive and noisy than smooth translation. But intuition is often wrong for small Reynolds number phenomena.
Swimming microorganisms
Back to list of research areas